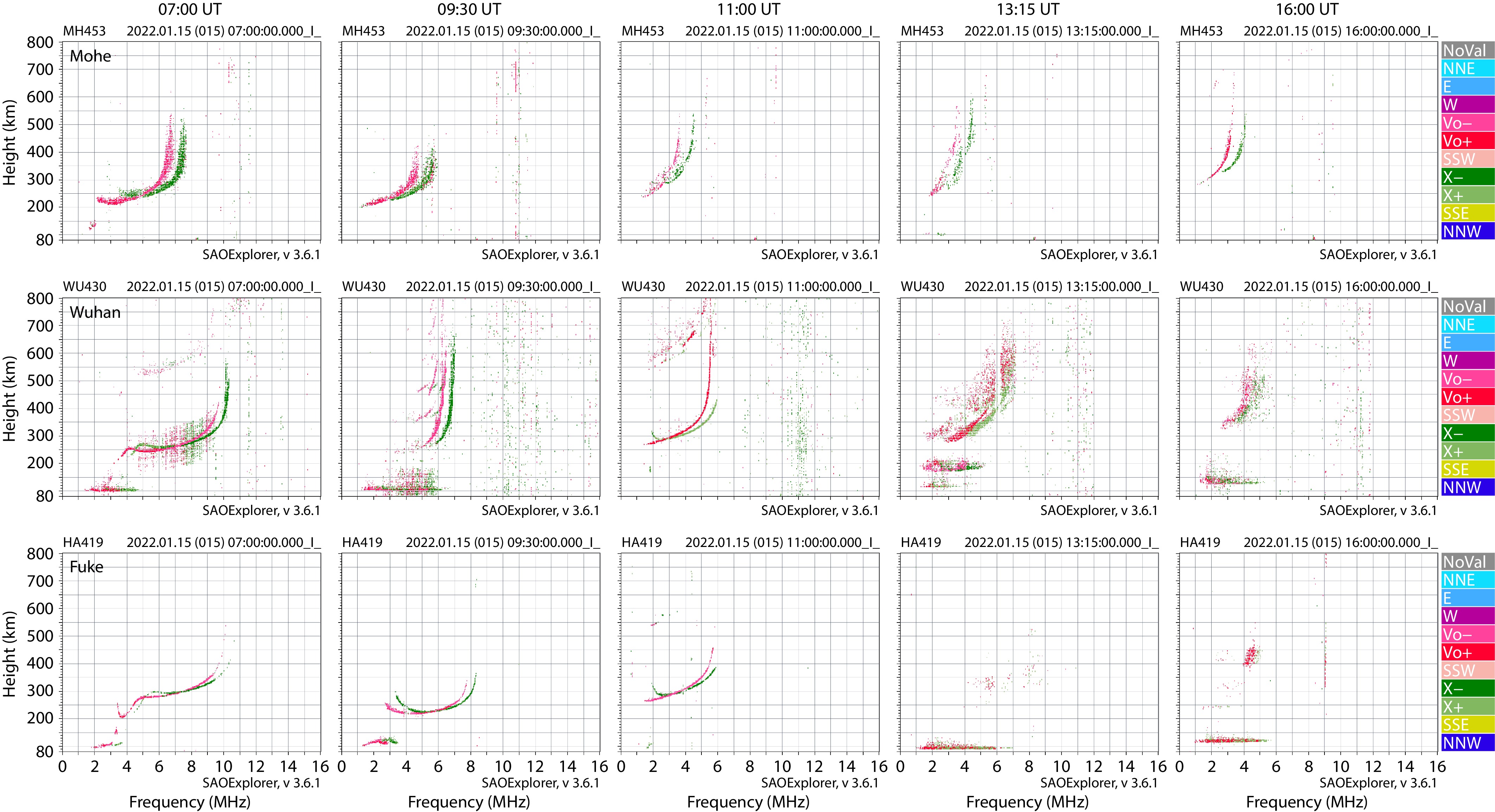
Citation: | Sun, Y. Y., Yu, T., Wang, J., and Long, C. (2025). Sporadic E responds to the 2022 Tonga volcano eruptions recorded by the Meridian Project. Earth Planet. Phys., 9(1), 20–28. DOI: 10.26464/epp2024051 |
In this study, ionosonde observations over Fuke (19.5°N, 109.1°E), Wuhan (30.5°N, 114.4°E), and Mohe (53.5°N, 122.3°E) were analyzed to demonstrate the responses of the sporadic E () to the severe atmospheric disturbances caused by the Tonga volcanic eruptions on January 15, 2022. The most prominent signature was the disappearance of the layer after ~10:00 UT over Wuhan and Fuke, which was attributed to the vertical drift caused by the eruptions. The occurred intermittently after 13:00 UT following the arrival of the tropospheric Lamb wave. To examine the causal mechanism for the intermittence, we also included data of horizontal winds in the mesosphere and lower thermosphere region recorded by the meteor radars at Wuhan and Mohe in this study. The wind disturbances with periods of ~20 hours contributed to the formation of the layer in the nighttime on January 15.
Numerous studies have demonstrated that eruptions of the underwater volcano near the Hunga Tonga-Hunga Ha’apai Island (20.55°S, 175.39°W), Kingdom of Tonga, after 04:10 universal time (UT) on January 15, 2022, triggered significant atmospheric and ionospheric disturbances, with the majority of the research focusing on the effects in the ionospheric F region (Chen CH et al., 2023; Shinbori et al., 2023, and references in). This event led to several notable phenomena, including interhemispheric electrodynamic coupling, substantial E × B drifts, and extreme plasma bubbles in the F region (e.g., Aa et al., 2022; Gasque et al., 2022; Lin JT et al., 2022; Rajesh et al., 2022; Shinbori et al., 2022; Sun WJ et al., 2022; Huba et al., 2023), marking new discoveries. The eruptions caused global disturbances of the electronic current in the ionospheric E region at ~100 km altitude (Liu JY et al., 2023). However, the response of the E-region density structure, such as sporadic E (), to the eruptions has not been investigated.
This study presents findings on the eruption-induced disturbances in , as observed by three ionosondes located at Fuke (19.5°N, 109.1°E), Hainan Province; Wuhan (30.5°N, 114.4°E), Hubei Province; and Mohe (53.5°N, 122.3°E), Heilongjiang Province. The ionosondes recorded notable plasma drifts subsequent to the eruptions on January 15 (Sun WJ et al., 2022; Qiu SC et al., 2023), despite being nearly 10,000 km distant from the Tonga volcano. On the other hand, previous studies have shown significant disturbances in the mesosphere and lower thermosphere (MLT) region after the eruptions (Harding et al., 2022; Liu X et al., 2022). Wind disturbances with periods of ~2 to 3 hours in the MLT region were recorded by meteor radars (Poblet et al., 2023; Stober et al., 2023). Therefore, we also included the horizontal wind observations as recorded by meteor radars in Wuhan and Mohe (Yu Y et al., 2013; Liu LB et al., 2017) to examine the potential mechanisms behind the disturbances.
The ionosondes and meteor radars are operated by the Meridian Project, which is a ground-based network program aimed at monitoring and investigating the solar–terrestrial space environment since 2012. The project comprises more than thirty stations and near three hundred instruments situated along longitudes of ~100°–120°E, and latitudes of ~30°–40°N (https://data.meridianproject.ac.cn). This collaborative effort involves more than ten institutions or universities in China, spearheaded by the National Space Science Center of the Chinese Academy of Sciences (Wang C et al., 2022).
An ionosonde is a radar system that automatically transmits electromagnetic waves upward into the ionosphere and captures the echoes reflected back to Earth. This process enables it to gather data on the density structure of the ionosphere at various altitudes. The data, presented as an ionogram, displays these echoes as a function of altitude and frequency. The ionosondes at Wuhan and Mohe recorded the ionograms every 15 minutes. The temporal resolution for the ionograms recorded at Fuke is 5 minutes. This study focuses on the ordinary-mode high-frequency echoes between 80 and 200 km altitudes to study the behaviors. We also converted the ionograms into height-time-amplitude (HTA) contours to show the temporal evolution of the layer. The HTA is the integration of the echo amplitude over the sounding frequencies ranging from 1.0 to 15.0 MHz at each altitude from each ionogram, which shows the total echo amplitude as a function of the virtual height and UT (Wang J et al., 2021).
Meteor radar measures neutral winds in the MLT region by utilizing the reflection of electromagnetic waves from meteor trails. The temporal resolution for the horizontal winds observed by the meteor radars over Wuhan and Mohe is 1 hour from 70 to 110 km altitudes (Yu Y et al., 2013; Liu LB et al., 2017). Note that data gaps occur for the ionosonde data over Beijing after 10:00 UT. Therefore, we did not include the data over Beijing in this study. The wind data over Hainan in 2022 are unavailable. The Hilbert−Huang transform is an efficient time−frequency analysis method, designed to identify changes in non-stationary and nonlinear disturbances within data sets. This transform computes the instantaneous frequency (f) and amplitude from a time series. The amplitudes are then averaged at each frequency−time (f−t) grid to create the Hilbert spectrum, H(f, t). The spectrum demonstrates the temporal and frequency evolution (Sun YY et al., 2015, 2021) of wind disturbances following the eruptions.
Figure 1 presents ionogram samples over Mohe, Wuhan, and Fuke, captured at 07:00, 09:30, 11:00, 13:15, and 16:00 UT on January 15, 2022. The ionograms at 07:00 UT for Wuhan and Fuke illustrate well-defined F and E layers, indicating stability. By 09:30 UT, the ionograms exhibit a noticeable strengthening of the layer. At 11:00 UT, the ionograms depict an uplifted F layer, coinciding with the disappearance of the layer. The ionograms at 13:15 UT reveal the occurrence of spread F and the return of the layer. By 16:00 UT, the ionograms display the presence of the layer in the nighttime. Overall, the ionograms over Mohe (top panels) demonstrate significantly weaker E-region echoes compared with those over Wuhan (middle panels) and Fuke (bottom panels).
Figure 2 illustrates the HTA contours for ionograms at altitudes ranging from 80 to 600 km over Fuke, Wuhan, and Mohe from January 14 to 16, 2022. A sudden uplift of the F layer occurred over Fuke and Wuhan after ~09:30 UT, which revealed the vertical drift that was reported by numerous studies (e.g., Aa et al., 2022; Rajesh et al., 2022; Sun WJ et al., 2022; Qiu SC et al., 2023). It is a large-scale ionospheric disturbance caused by the eruption (e.g., Chen CH et al., 2022; Heki, 2022; Sun WJ et al., 2022). The spread-F echoes appeared during the periods of ~12:45–16:00 UT and ~13:00–15:00 UT over Fuke and Wuhan, respectively, which indicated the occurrence of plasma irregularities in the F region caused by the eruptions (e.g., Aa et al., 2022; Rajesh et al., 2022; Sun WJ et al., 2022; Huba et al., 2023). The echoes over Mohe are weaker than those over Wuhan and Fuke. Both the F and E layers were disturbed after ~10:00 UT over Fuke and Wuhan (Figures 2b and 2e), which corresponds to the ionospheric disturbance with a velocity of ~450 m/s. The velocity was calculated by dividing the distance between the station and the Tonga volcano by the time difference between the occurrence of the disturbance and the eruption.
Figure 3 illustrates the HTA plots for the echo intensity below 200 km altitude over the three stations. In Figure 3a, the layer occurred mainly in the daytime and no occurred after 11:00 UT in the nighttime over Fuke on January 14, which is one day before the eruption day. In Figure 3b, the layer is stable before 10:00 UT, but it is intermittent in the nighttime on January 15. We indicated the arrival time of the tropospheric Lamb wave with a velocity of 310 m/s (e.g., Amores et al., 2022; Chen CH et al., 2022; Yuen et al., 2022). The layer occurred from ~12:50 to ~14:00 UT following the arrival of the Lamb wave (Figure 3b). The E-region echoes appeared again and were disturbed between 80 and 140 km from 15:30 to 21:00 UT. The layer is weak on January 16 (Figure 3c).
The layer is intense in the daytime but vanished after 14:00 UT over Wuhan one day before the eruption day (Figure 3d). The layer also disappeared suddenly after 10:00 UT on January 15 (Figure 3e). The echoes from the layer appeared again over Wuhan after 15:00 UT and persisted throughout the entire nighttime. In Figure 3f, the layer is intense in the daytime but vanished in the nighttime on January 16, one day after the eruption. Overall, the echoes are weak over Mohe from January 14 to 16 (right column of Figure 3).
To examine the causal mechanism for the intermittence in the nighttime on the eruption day, we further analyzed the MLT wind recorded by the meteor radars at Wuhan and Mohe from January 14 to 16, 2022 (Figures 4 and 5). In Figure 4, the most prominent signature is the northward wind that occurred at 87 to 103 km altitudes over Wuhan from ~13:00 to ~20:00 UT on January 15 (Figure 4a). The wind disturbance reaches 60 m/s. In Figure 4b, the westward and eastward wind disturbances are also notable near 12:00 and 19:00 UT on January 15. The wind disturbance is not obvious over Mohe (Figure 5).
Figures 6 and 7 provide insights into the wind disturbances caused by the eruption, when the wind speeds averaged between altitudes of 90 and 100 km on January 15 were compared with the January monthly mean and showcasing their dynamic spectra. The top panels of Figure 6 reveal that, over Wuhan, the northward wind surpasses the error margin of the monthly mean (one standard deviation) from 13:00 to 23:00 UT, peaking at ~15:00 UT. Meanwhile, a westward wind disturbance falls below the error margin from 10:00 to 14:00 UT and climbs above it around 19:00 UT. The lower panels of Figure 6 illustrate the Hilbert spectral analysis of the averaged wind speeds, indicating that the winds exhibiting a ~20-hour period intensify after ~10:00 UT. Figure 7 illustrates that the wind disturbances over Mohe are less pronounced than those over Wuhan, as shown in Figure 6. The Hilbert spectra reveal a shift in the periodicity of the wind disturbances, evolving from approximately 10 hours to ~3 to 4 hours after ~10:00 UT.
The sudden disappearance of the layer over Wuhan and Fuke after ~10:00 UT corresponds to the vertical drift that began after ~09:30 UT (e.g., Sun WJ et al., 2022). This phenomenon mirrors the diminished strength of the layer observed over Chinese continent during the solar eclipse on June 21, 2020 (Wang J et al., 2021). The underlying cause was attributed to the upward drift (Wang J et al., 2022) caused by the eastward electric field, which has the effect of weakening the layer (Abdu et al., 2013; Resende et al., 2020). The results also indicated that strong electrodynamical changes caused large-scale ionospheric disturbances after the Tonga eruptions (Chen CH et al., 2023; Shinbori et al., 2023, and references in). The echo descended and an layer occurred over Wuhan and Fuke after ~15:00 UT (Figure 3), which is also similar to the occurrence of the descending echoes after the maximum obscuration of the 2020 solstice eclipse (Wang J et al., 2021). The strong spread F and began around 13:00 UT over Fuke and Wuhan (Figures 1 to 3), following the arrival of the tropospheric Lamb wave with a velocity of ~310 m/s (e.g., Amores et al., 2022; Chen CH et al., 2022; Yuen et al., 2022). This indicates that the leakage of the tropospheric Lamb wave (Chen CH et al., 2023) acts as a seeding mechanism for both the and spread F. The ionogram over Wuhan at 13:15 UT (Figure 1) shows the reoccurrence of Es. The reoccurring over Wuhan is weaker compared with that over Fuke following the arrival of the Lamb wave (Figures 3b and 3e). The spatial distribution of metallic ions is complex in the ionosphere (Huba et al., 2019), particularly during the disturbance period, which may cause the difference between the behaviors over the two locations, Wuhan and Fuke.
The reoccurrence of at ~15:00 UT (Figure 3) coincides with the significant disturbance in the MLT wind at ~15:00 UT recorded over Wuhan (Figure 6). It is surprising to observe the wind disturbances over Wuhan with periods around 20 hours (Figures 6b and 6d), which fall within the atmospheric tidal scale regime and are much longer than the previous results of long-period disturbances (periods of several hours) in the E region (Sun YY et al., 2022). Tidal winds are capable of triggering the formation of the layer through the process of wind-shear convergence (Shinagawa et al., 2017; Qiu LH et al., 2021). Note that the wind profile data in the lower thermosphere are unavailable for the wind-shear analysis here. The MIGHTI/ICON (Michelson Interferometer for Global High-resolution Thermospheric Imaging/Ionospheric Connection Explorer) observed the wind reversals between 100 and 150 km altitudes over the American-Atlantic area (Harding et al., 2022), which is evidence for the existence of wind shear attributable to the eruption-induced wind disturbances in the MLT region.
Conversely, the less pronounced wind disturbances with shorter periods of ~3 hours over Mohe may explain the lack of noticeable responses in that region. The broadband wind disturbances (Figures 6 and 7) hint at intricate interactions of atmospheric disturbances (Liu HL et al., 2023) and mean flows that happened from the lower atmosphere to the MLT region (Sun YY et al., 2018). These complicated phenomena warrant a detailed investigation to understand their dynamics and implications fully.
Spreads of the echoes occurred from 110 to 160 km altitude at ~06:00 to ~10:00 UT over the three ionosonde stations on January 16 (bottom panels of Figure 3), which mainly meets the backward disturbances that propagate along the long-path direction with a speed of 310 m/s over China around 07:00 UT (Li T et al., 2023). Such types of unique disturbances in the ionosonde echoes, which appear from the E layer to the bottom of the F layer, have also been observed because of the acoustic-gravity waves triggered by the 2011 Tohoku-oki earthquake and tsunami (Liu JY and Sun YY, 2011; Maruyama et al., 2011). These disturbances warrant further investigation. Short-period gravity waves can also contribute to the formation of the layer (Hock and Tsuda, 2001; Didebulidze et al., 2020), but the physical processes and mechanisms of gravity wave modulation on the layer require further revelation and study. The wind data with one-hour resolution cannot reveal the gravity waves with periods of minutes that may contribute to the echo spreading one day after the eruptions. The winds with high temporal resolution recorded by meteor radars in the MLT region (Long C et al., 2023; Stober et al., 2023) can be further used to investigate the gravity waves with shorter periods because of the eruptions and their effect on the layer.
This study marks the first exploration into how atmospheric disturbances attributable to the Tonga volcano eruptions on January 15, 2022, influenced the disappearance and reoccurrence of the layer. The eruption-induced vertical drift led to the layer vanishing after ~10:00 UT over Wuhan and Fuke. The significant wind disturbances in the MLT region, reaching speeds of ~60 m/s and periods of ~20 hours, triggered the reoccurrence of the layer over Fuke and Wuhan during the night (after 15:00 UT). The propagation of a tropospheric Lamb wave, moving at a velocity of ~310 m/s, seeded the and spread F after ~13:00 UT. The results in this study suggest that plasma instabilities in both the ionospheric F and E regions, spurred by significant atmospheric disturbances from below, including events of terrestrial activities, such as volcanic eruptions, earthquakes, tsunamis, cyclones, and strong convections, can be delved into deeper in the future.
We acknowledge the Chinese Meridian Project for sharing the data of ionosondes and meteor radars (https://data.meridianproject.ac.cn/).
This research was funded by the Funds of the National Natural Science Foundation of China (NSFC), grant numbers
Aa, E., Zhang, S. R., Erickson, P. J., Vierinen, J., Coster, A. J., Goncharenko, L. P., Spicher, A., and Rideout, W. (2022). Significant ionospheric hole and equatorial plasma bubbles after the 2022 Tonga volcano eruption. Space Wea., 20(7), e2022SW003101. https://doi.org/10.1029/2022SW003101
|
Abdu, M. A., Souza, J. R., Batista, I. S., Fejer, B. G., and Sobral. J. H. A. (2013). Sporadic E layer development and disruption at low latitudes by prompt penetration electric fields during magnetic storms. J. Geophys. Res.: Space Phys., 118(5), 2639–2647. https://doi.org/10.1002/jgra.50271
|
Amores, A., Monserrat, S., Marcos, M., Argüeso, D., Villalonga, J., Jordà, G., and Gomisà, D. (2022). Numerical simulation of atmospheric Lamb waves generated by the 2022 Hunga-Tonga volcanic eruption. Geophys. Res. Lett., 49(6), e2022GL098240. https://doi.org/10.1029/2022GL098240
|
Chen, C. H., Zhang, X. M., Sun, Y. Y., Wang, F., Liu, T. C., Lin, C. Y., Gao, Y. X., Lyu, J., Jin, X. B., … Liu, J. Y. (2022) Individual wave propagations in ionosphere and troposphere triggered by the Hunga Tonga-Hunga Ha’apai underwater volcano eruption on 15 January 2022. Remote Sens., 14(9), 2179. https://doi.org/10.3390/rs14092179
|
Chen, C. H., Sun, Y. Y., Zhang, X. M., Wang, F., Lin, K., Gao, Y. X., Tang, C. C., Lyu, J., Huang, R., and Huang, Q. (2023). Far-field coupling and interactions in multiple geospheres after the Tonga volcano eruptions. Surv. Geophys., 44(3), 587–601. https://doi.org/10.1007/s10712-022-09753-w
|
Didebulidze, G. G., Dalakishvili, G., and Todua, M. (2020). Formation of multilayered sporadic E under an influence of atmospheric gravity waves (AGWs). Atmosphere, 11(6), 653. https://doi.org/10.3390/atmos11060653
|
Gasque, L. C., Wu, Y. J., Harding, B. J., Immel, T. J., and Triplett, C. C. (2022). Rapid volcanic modification of the E-region dynamo: ICON's first glimpse of the Tonga eruption. Geophys. Res. Lett., 49(18), e2022GL100825. https://doi.org/10.1029/2022GL100825
|
Harding, B. J., Wu, Y. J. J., Alken, P., Yamazaki, Y., Triplett, C. C., Immel, T. J., Gasque, L. C., Mende, S. B., and Xiong, C. (2022). Impacts of the January 2022 Tonga volcanic eruption on the ionospheric dynamo: ICON-MIGHTI and swarm observations of extreme neutral winds and currents. Geophys. Res. Lett., 49(9), e2022GL098577. https://doi.org/10.1029/2022GL098577
|
Heki, K. (2022). Ionospheric signatures of repeated passages of atmospheric waves by the 2022 Jan. 15 Hunga Tonga-Hunga Ha’apai eruption detected by QZSS-TEC observations in Japan. Earth Planets Space, 74(1), 112. https://doi.org/10.1186/s40623-022-01674-7
|
Hocke, K., and Tsuda, T. (2001). Gravity waves and ionospheric irregularities over tropical convection zones observed by GPS/MET radio occultation. Geophys. Res. Lett., 28(14), 2815–2818. https://doi.org/10.1029/2001GL013076
|
Huba, J. D., Krall, J., and Drob, D. (2019). Global ionospheric metal ion transport with SAMI3. Geophys. Res. Lett., 46(14), 7937–7944. https://doi.org/10.1029/2019GL083583
|
Huba, J. D., Becker, E., and Vadas, S. L. (2023). Simulation study of the 15 January 2022 Tonga event: Development of super equatorial plasma bubbles. Geophys. Res. Lett., 50(1), e2022GL101185. https://doi.org/10.1029/2022GL101185
|
Li, T., Gao, Y. X., Chen, C. H., Zhang, X. M., and Sun, Y. Y. (2023). Ionospheric disturbances observed over China after 2022 January 15 Tonga volcano eruption. Geophys. J. Int., 235(1), 909–919. https://doi.org/10.1093/gji/ggad273
|
Lin, J. T., Rajesh, P. K., Lin, C. C. H., Chou, M. Y., Liu, J. Y., Yue, J., Hsiao, T. Y., Tsai, H. F., Chao, H. M., and Kung, M. M. (2022). Rapid conjugate appearance of the giant ionospheric Lamb wave signatures in the northern hemisphere after Hunga-Tonga volcano eruptions. Geophys. Res. Lett., 49(8), e2022GL098222. https://doi.org/10.1029/2022GL098222
|
Liu, H. L., Wang, W., Huba, J. D., Lauritzen, P. H., and Vitt, F. (2023). Atmospheric and ionospheric responses to Hunga-Tonga volcano eruption simulated by WACCM-X. Geophys. Res. Lett., 50(10), e2023GL103682. https://doi.org/10.1029/2023GL103682
|
Liu, J. Y., and Sun, Y. Y. (2011). Seismo-traveling ionospheric disturbances of ionograms observed during the 2011 Mw 9.0 Tohoku Earthquake. Earth Planets Space, 63(7), 70. https://doi.org/10.5047/eps.2011.05.017
|
Liu, J. Y., Kao, T. H., Liu, T. C., Huang, B. S., Lee, P. H., Sun, Y. Y., Chen, C. H., Hattori, K., Liao, P. H., … Huang, T. S. (2023). Magnetic field signatures of tropospheric and thermospheric Lamb modes triggered by the 15 January 2022 Tonga volcanic eruption. Geophys. Res. Lett., 50(20), e2023GL105393. https://doi.org/10.1029/2023GL105393
|
Liu, L. B., Liu, H. X., Chen, Y. D., Le, H. J., Sun, Y. Y., Ning, B. Q., Hu, L. H., and Wan, W. X. (2017). Variations of the meteor echo heights at Beijing and Mohe, China. J. Geophys. Res.: Space Phys., 122(1), 1117–1127. https://doi.org/10.1002/2016JA023448
|
Liu, X., Xu, J. Y., Yue, J., and Kogure, M. (2022). Strong gravity waves associated with Tonga volcano eruption revealed by SABER observations. Geophys. Res. Lett., 49(10), e2022GL098339. https://doi.org/10.1029/2022GL098339
|
Long, C., Yu, T., Sun, Y. Y., Yan, X. X., Zhang, J., Yang, N., Wang, J., Xia, C. L., Liang, Y., and Ye, H. L. (2023). Atmospheric gravity wave derived from the neutral wind with 5-minute resolution routinely retrieved by the meteor radar at Mohe. Remote Sens., 15(2), 296. https://doi.org/10.3390/rs15020296
|
Maruyama, T., Tsugawa, T., Kato, H., Saito, A., Otsuka, Y., and Nishioka, M. (2011). Ionospheric multiple stratifications and irregularities induced by the 2011 off the Pacific coast of Tohoku earthquake. Earth Planets Space, 63(7), 869–873. https://doi.org/10.5047/eps.2011.06.008
|
Poblet, F. L., Chau, J. L., Conte, J. F., Vierinen, J., Suclupe, J., Liu, A., and Rodriguez, R. R. (2023). Extreme horizontal wind perturbations in the mesosphere and lower thermosphere over South America associated with the 2022 Hunga eruption. Geophys. Res. Lett., 50(12), e2023GL103809. https://doi.org/10.1029/2023GL103809
|
Qiu, L. H., Zuo, X. M., Yu, T., Sun, Y. Y., Liu, H. X., Sun, L. F., and Zhao, B. Q. (2021). The characteristics of summer descending sporadic E layer observed with the ionosondes in the China region. J. Geophys. Res.: Space Phys., 126(3), e2020JA028729. https://doi.org/10.1029/2020JA028729
|
Qiu, S. C., Shi, M. X., Wang, X. Y., Zhang, Z. M., Soon, W., and Herrera, V. M. V. (2023). An investigation on the ionospheric response to the volcanic explosion of Hunga Ha’apai, 2022, based on the observations from the Meridian Project: The plasma drift variations. Remote Sens., 15(17), 4181. https://doi.org/10.3390/rs15174181
|
Rajesh, P. K., Lin, C. C. H., Lin, J. T., Lin, C. Y., Liu, J. Y., Matsuo, T., Huang, C. Y., Chou, M. Y., Yue, C. J., and Tsai, H. F. (2022). Extreme poleward expanding super plasma bubbles over Asia-Pacific region triggered by Tonga volcano eruption during the recovery-phase of geomagnetic storm. Geophys. Res. Lett., 49(15), e2022GL099798. https://doi.org/10.1029/2022GL099798
|
Resende, L. C. A., Shi, J. K., Denardini, C. M., Batista, I. S., Nogueira, P. A. B., Arras, C., Andrioli, V. F., Moro, J., Da Silva, L.A., and Liu, Z. (2020). The influence of disturbance dynamo electric field in the formation of strong sporadic E layers over Boa Vista, a low-latitude station in the American sector. J. Geophys. Res.: Space Phys., 125(7), e2019JA027519. https://doi.org/10.1029/2019JA027519
|
Shinagawa, H., Miyoshi, Y., Jin, H., and Fujiwara, H. (2017). Global distribution of neutral wind shear associated with sporadic E layers derived from GAIA. J. Geophys. Res.: Space Phys., 122(4), 4450–4465. https://doi.org/10.1002/2016JA023778
|
Shinbori, A., Otsuka, Y., Sori, T., Nishioka, M., Perwitasari, S., Tsuda, T., and Nishitani, N. (2022). Electromagnetic conjugacy of ionospheric disturbances after the 2022 Hunga Tonga-Hunga Ha’apai volcanic eruption as seen in GNSS-TEC and SuperDARN Hokkaido pair of radars observations. Earth Planets Space, 74(1), 106. https://doi.org/10.1186/s40623-022-01665-8
|
Shinbori, A., Otsuka, Y., Sori, T., Nishioka, M., Septi, P., Tsuda, T., Nishitani, N., Kumamoto, A., Tsuchiya, F., … Shinohara, I. (2023). New aspects of the upper atmospheric disturbances caused by the explosive eruption of the 2022 Hunga Tonga–Hunga Ha’apai volcano. Earth Planets Space, 75(1), 175. https://doi.org/10.1186/s40623-023-01930-4
|
Stober, G., Liu, A., Kozlovsky, A., Qiao, Z. S., Krochin, W., Shi, G. C., Kero, J., Tsutsumi, M., Gulbrandsen, N., … Mitchell, N. (2023). Identifying gravity waves launched by the Hunga Tonga-Hunga Ha’apai volcanic eruption in mesosphere/lower-thermosphere winds derived from CONDOR and the Nordic Meteor Radar Cluster. Ann. Geophys., 41(1), 197–208. https://doi.org/10.5194/angeo-41-197-2023
|
Sun, W. J., Kuriakose, A. K., Li, G. Z., Li, Y., Zhao, X. K., Hu, L. H., Yang, S. P., Xie, H. Y., Li, Y., … Liu, L. B. (2022). Unseasonal super ionospheric plasma bubble and scintillations seeded by the 2022 Tonga Volcano Eruption related perturbations. J. Space Wea. Space Clim., 12, 25. https://doi.org/10.1051/swsc/2022024
|
Sun, Y. Y., Chen, C. H., Liu, J. Y., Wang, C. H., and Chen, D. L. (2015). Instantaneous phase shift of annual subsurface temperature cycles derived by the Hilbert-Huang transform. J. Geophys. Res.: Atmos., 120(4), 1670–1677. https://doi.org/10.1002/2014JD022574
|
Sun, Y. Y., Liu, H. X., Miyoshi, Y., Liu, L. B., and Chang, L. C. (2018). El Niño-Southern Oscillation effect on quasi-biennial oscillations of temperature diurnal tides in the mesosphere and lower thermosphere. Earth Planets Space, 70(1), 85. https://doi.org/10.1186/s40623-018-0832-6
|
Sun, Y. Y., Shen, M. M., Tsai, Y. L., Lin, C. Y., Chou, M. Y., Yu, T., Lin, K., Huang, Q., Wang, J., … Liu, J. Y. (2021). Wave steepening in ionospheric total electron density due to the 21 August 2017 total solar eclipse. J. Geophys. Res.: Space Phys., 126(3), e2020JA028931. https://doi.org/10.1029/2020JA028931
|
Sun, Y. Y., Chen, C. H., Zhang, P., Li, S., Xu, H. R., Yu, T., Lin, K., Mao, Z. Q., Zhang, S. X., … Liu, J. Y. (2022). Explosive eruption of the Tonga underwater volcano modulates the ionospheric E-region current on 15 January 2022. Geophys. Res. Lett., 49(15), e2022GL099621. https://doi.org/10.1029/2022GL099621
|
Wang, C., Xu, J. Y., Lü, D. R., Yue, X. N., Xue, X. H., Chen, G., Yan, J. Y., Yan, Y. H., Lan, A. L., … Tian, Y. F. (2022). Construction progress of Chinese Meridian Project Phase II. Chin. J. Space Sci., 42(4), 539–545. https://doi.org/10.11728/cjss2022.04.yg09
|
Wang, J., Zuo, X. M., Sun, Y. Y., Yu, T., Wang, Y. G., Qiu, L. H., Mao, T., Yan, X. X., Yang, N., … Zhao, B. Q. (2021). Multilayered sporadic-E response to the annular solar eclipse on June 21, 2020. Space Wea., 19(3), e2020SW002643. https://doi.org/10.1029/2020SW002643
|
Wang, J., Sun, Y. Y., Yu, T., Wang, Y. G., Mao, T., Yang, H., Xia, C. L., Yan, X. X., Yang, N., … Qi, Y. F. (2022). Convergence effects on the ionosphere during and after the annular solar eclipse on 21 June 2020. J. Geophys. Res.: Space Phys., 127(9), e2022JA030471. https://doi.org/10.1029/2022JA030471
|
Yu, Y., Wan, W. X., Ning, B. Q., Liu, L. B., Wang, Z. G., Hu, L. H., and Ren, Z. P. (2013). Tidal wind mapping from observations of a meteor radar chain in December 2011. J. Geophys. Res.: Space Phys., 118(5), 2321–2332. https://doi.org/10.1029/2012JA017976
|
Yuen, D. A., Scruggs, M. A., Spera, F. J., Zheng, Y. C., Hu, H., McNutt, S. R., Thompson, G., Mandli, K., Keller, B. R., … Tanioka, Y. (2022). Under the surface: Pressure-induced planetary-scale waves, volcanic lightning, and gaseous clouds caused by the submarine eruption of Hunga Tonga-Hunga Ha’apai volcano. Earthq. Res. Adv., 2(3), 100134. https://doi.org/10.1016/j.eqrea.2022.100134
|
WenYu Hao, Tao Yu, Yang-Yi Sun, Jin Wang, LiHui Qiu. 2025: Morphology and dynamics of sporadic E layers observed by amateur radio. Earth and Planetary Physics, 9(4): 1-8. DOI: 10.26464/epp2025019 | |
Siti Syazwani Nasuha, Idahwati Sarudin, Nurul Shazana Abdul Hamid, Ahmad Fairuz Omar. 2025: Thermospheric neutral wind studies over the equatorial region: A review. Earth and Planetary Physics, 9(1): 81-100. DOI: 10.26464/epp2024074 | |
JiaWei Wu, Chao Xiong, YuYang Huang, YunLiang Zhou. 2025: Vertical gradients of neutral winds observed by ICON and estimated by the Horizontal Wind Model during the geomagnetic storm on August 26−28, 2021. Earth and Planetary Physics, 9(1): 69-80. DOI: 10.26464/epp2024033 | |
BingKun Yu, PengHao Tian, XiangHui Xue, Christopher J. Scott, HaiLun Ye, JianFei Wu, Wen Yi, TingDi Chen, XianKang Dou. 2025: Comparative analysis of empirical and deep learning models for ionospheric sporadic E layer prediction. Earth and Planetary Physics, 9(1): 10-19. DOI: 10.26464/epp2024048 | |
Xu Zhou, ZeZhong Li, XinAn Yue, LiBo Liu. 2025: Effects of mesoscale gravity waves on sporadic E simulated by a one-dimensional dynamic model. Earth and Planetary Physics, 9(1): 1-9. DOI: 10.26464/epp2024038 | |
Dandan Li, Jun Liu, Weifeng Hao, Sergey Popov, Lin Li, Fei Li, Xiangbin Cui, Shinan Lang, Bo Sun, and Martin Siegert. 2025: Physiography and basal melt rate of subglacial Lake 90°E. Earth and Planetary Physics, 9(0). DOI: 10.26464/epp2025069 | |
YuChang Xun, ZhiShan Chen, LiFang Du, HaoRan Zheng, Jing Jiao, ZeLong Wang, ShaoHua Gong, GuoTao Yang. 2024: A statistical study on the correlation between sporadic Ca+ layer and Es in Beijing (40.5°N, 116°E). Earth and Planetary Physics, 8(5): 753-761. DOI: 10.26464/epp2024046 | |
LiHui Qiu, Xian Lu, Tao Yu, Yosuke Yamazaki, HuiXin Liu, Yang-Yi Sun, HaoNan Wu, XiaoMin Zuo, XiangXiang Yan, Yan Yu, YiFan Qi. 2023: Horizontal structure of convergent wind shear associated with sporadic E layers over East Asia. Earth and Planetary Physics, 7(5): 548-557. DOI: 10.26464/epp2023071 | |
P. Abadi, Y. Otsuka, HuiXin Liu, K. Hozumi, D. R. Martinigrum, P. Jamjareegulgarn, Le Truong Thanh, R. Otadoy. 2021: Roles of thermospheric neutral wind and equatorial electrojet in pre-reversal enhancement, deduced from observations in Southeast Asia. Earth and Planetary Physics, 5(5): 387-396. DOI: 10.26464/epp2021049 | |
YaLi Wang, Tao Xie, YanRu An, Chong Yue, JiuYang Wang, Chen Yu, Li Yao, Jun Lu. 2019: Characteristics of the coseismic geomagnetic disturbances recorded during the 2008 Mw 7.9 Wenchuan Earthquake and two unexplained problems. Earth and Planetary Physics, 3(5): 435-443. DOI: 10.26464/epp2019043 |